Anesthesiology News
Definition of General Anesthesia
“General anesthesia” is a drug-induced reversible behavioral state consisting of antinociception, unconsciousness, amnesia and akinesia with maintenance of physiologic systems stability.1,2When anesthesiology was first practiced, the definition was not as clear as stated here, and a single agent, namely ether, was relied upon to produce the state that we now call general anesthesia.
Today, balanced general anesthesia is one of the most common management approaches to delivering general anesthesia.3 It consists of using different drugs to achieve at least approximately each of the separate characteristics of the anesthetic state: for example, opioids to produce antinociception, propofol or sevoflurane to produce unconsciousness and amnesia, and rocuronium or other neuromuscular blocking agents to achieve akinesia. Balanced general anesthesia is predicated on the idea that using combinations of agents makes it possible to use less of each drug and thereby achieve more of the desired effects and fewer of the undesired side effects.4,5
Multimodal General Anesthesia
Multimodal general anesthesia builds on the practices established in balanced general anesthesia and, at least in the United States, is also motivated by the opioid crisis.2 To explain multimodal general anesthesia, we must first draw the distinction between nociception and pain. Nociception is the transmission through the central nervous system of responses to noxious and likely harmful stimuli, whereas pain is the conscious perception of nociceptive information.2During surgery, when the patient is unconscious, analgesic medications are given to control nociception, whereas during the postoperative period, when the patient has recovered consciousness, analgesics are administered to treat pain.
There are four basic ideas involved in multimodal general anesthesia. First, multiple agents are administered to control nociception during surgery (Table 1). The combination of drugs is chosen so that each drug acts on a different component of the nociceptive (pain) pathways (Table 2). This suggests a better way to control nociception intraoperatively and pain postoperatively.
Table 1. Multimodal General Anesthesia: Explicit and Implicit Maintenance Of the Anesthetic State | ||
Anesthetic Statea | Explicit Maintenanceb | Implicit Maintenancec |
---|---|---|
Antinociception |
|
|
Unconsciousness (amnesia) |
|
|
Immobility |
|
|
a Defines the behavioral component of general anesthesia.
b Examples of anesthetic agents that can be used to maintain the behavioral state of general anesthesia.
c Additional behavioral states to which the anesthetic contributes.
GABA, gamma-aminobutyric acid; NMDA, N-methyl-D-aspartate; NSAID, nonsteroidal anti-inflammatory drug
Based on reference 2.
|
Table 2. Antinociceptive Agents: Mechanisms of Antinociception, Sedation And Unconsciousness | ||
Agent | Mechanism of Antinociception | Mechanism of Sedation and Unconsciousness |
---|---|---|
Opioids | Binding to opioid receptors blocks peripheral afferent nociceptive inputs into the spinal cord; enhances descending inhibition of nociceptive inputs beginning at the level of the periaqueductal gray in the brainstem; and decreases nociceptive perception and the emotional effect of nociceptive stimuli in the amygdala. | Multisite disruption of nociceptive information transmission decreases arousal. Opioids inhibit cholinergic arousal inputs from the brainstem (LDT, PPT, mPRF) into the thalamus. This likely induces hyperpolarization (creation of up–down states) in the thalamus and cortex. |
Ketamine (NMDA-receptor antagonist) | Binding to NMDA glutamate receptors on peripheral afferent neurons in the dorsal horn blocks entrance of nociceptive information into the spinal cord. Binding to NMDA receptors in the cortex and thalamus blocks intracortical, thalamocortical, brainstem-thalamic and brainstem-cortical communication, thus enhancing antinociception. Low ketamine doses favor inactivation of inhibitory interneurons, whereas higher doses inactivate both inhibitory and excitatory neurons. | Multisite disruption of nociceptive information transmission decreases arousal. Low ketamine doses are commonly associated with hallucinations. A key site of action is likely to be NMDA glutamate projections from the parabrachial nucleus into the basal forebrain and the central thalamus. |
Magnesium (NMDA-receptor antagonist) | The NMDA sites of action are likely similar to those of ketamine. | Decreased arousal is likely due to a general decrease in NMDA glutamate–mediated excitatory transmission in the spinal cord, brainstem, thalamus and cortex. This likely fosters hyperpolarization (creation of up–down states) in the thalamus and cortex. |
Dexmedetomidine (alpha-2 adrenergic-receptor agonist) | Binding to alpha-2 adrenergic receptors enhances descending inhibition of nociceptive transmission by activating inhibitory interneurons synapsing onto projection neurons in the spinal cord dorsal horn. Dexmedetomidine also blocks presynaptic release of norepinephrine from the locus ceruleus in the brainstem, and its arousal inputs to the hypothalamus, basal forebrain, cortex and central thalamus. | Dexmedetomidine’s antinociceptive actions in the spinal cord, hypothalamus, cortex, basal forebrain and central thalamus contribute directly to its sedative effects. This likely fosters hyperpolarization (creation of up–down states) in the thalamus and cortex. |
NSAIDs | Inhibiting COX-1 and COX-2 enzymes blocks the conversion of arachidonic acid to prostaglandins, thereby reducing the production of these important inflammatory and nociceptive mediators. | The effects of NSAIDs on decreasing arousal are likely indirect by reducing the contribution of inflammation to enhancing nociceptive transmission in the central nervous system. |
IV Lidocaine | By blocking neutrophil priming, IV lidocaine impairs the ability of these cells to amplify the inflammatory response and consequently nociceptive signaling. | Patients receiving IV lidocaine infusions often note a feeling of sedation. |
COX, cyclooxygenase; LDT, laterodorsal tegmental nucleus; mPRF, medial pontine reticular formation; NMDA, N-methyl-D-aspartate; NSAIDs, nonsteroidal anti-inflammatory drugs; PPT, pedunculopontine tegmentum
Based on reference 2.
|
Second, because of the close relationship between the nociceptive pathways and the arousal pathways, these antinociceptive agents also act in the arousal pathways, where they help decrease arousal (see Table 1, first row, second column).2 Their effects on arousals are seen in the fact that they contribute to making the patient unconscious. Therefore, the dose of the hypnotic anesthetic, such as a propofol infusion or the flow of an inhaled ether, required to maintain an appropriate level of unconsciousness is often greatly decreased (Table 1, second row, second column). The use of less propofol or sevoflurane to maintain unconsciousness suggests a strategy for reducing exposure to these agents and the potential side effects of delirium and postoperative cognitive dysfunction.
Third, to manage multimodal general anesthesia properly, the anesthesia care provider must have an explicit strategy for monitoring the level of antinociception and level of unconsciousness. To date, we use heart rate and blood pressure changes interpreted within the clinical context to infer level of antinociception.6 In our practice, we use a real-time display of the unprocessed EEG recordings and its spectrogram to infer the patient’s level of unconsciousness.7
Fourth, a multimodal strategy should also be followed postoperatively to manage the patient’s pain.2 In Table 1, we summarize the logic for choosing the different anesthetic combinations, stating their primary (explicit) and secondary (implicit) effects. In Table 2, we summarize for each of the principal analgesics their sites and mechanisms of action in the nociceptive pathways as well as their sites and mechanisms of action in the arousal pathways. In Table 3, we summarize for propofol and sevoflurane their sites and mechanisms of action in the arousal pathways in addition to how they affect nociception.
Table 3. Hypnotic Agents: Mechanisms of Sedation, Unconsciousness And Antinociception | ||
Hypnotic Agents | Mechanism of Sedation and Unconsciousness | Mechanism of Antinociception |
---|---|---|
Propofol (GABA agonist) | Propofol produces sedation and unconsciousness by enhancing GABAergic activity of inhibitory interneurons in the cortex, in the thalamus, at inhibitory GABAergic projections from the hypothalamus onto the brainstem arousal centers, and in the spinal cord. | Propofol contributes to antinociception primarily by decreasing perception of nociceptive stimuli by inducing states of sedation or unconsciousness. |
Inhaled ethers (GABA agonist) | These anesthetics produce sedation and unconsciousness by enhancing GABAergic activity of inhibitory interneurons in the cortex, in the thalamus, at inhibitory GABAergic projections from the hypothalamus onto the brainstem arousal centers, and in the spinal cord. These agents also inhibit several other ion channels and receptor targets. | These anesthetics contribute to antinociception primarily by decreasing perception of nociceptive stimuli by inducing states of sedation or unconsciousness. |
GABA, gamma-aminobutyric acid
Based on reference 2.
|
Case Studies
We illustrate the multimodal general anesthesia paradigm using the following set of case studies.
Case 1
A 54-year-old woman underwent a bilateral mastectomy with reconstruction. Her weight was 74.8 kg and height was 165 cm. The patient received an ultrasound-guided bilateral trunk-paravertebral block for intra- and postoperative pain management. Prior to the block procedure, she received 100 mcg of fentanyl and 2 mg of midazolam. For the block, she received bupivacaine 0.5% with epinephrine 1:400,000, administered as 15 mL on the right side and 20 mL on the left.
Approximately 30 minutes after placement of the block, general anesthesia was induced with IV administration of propofol 160 mg, lidocaine 60 mg, fentanyl 100 mcg, and rocuronium 50 mg.
For maintenance of general anesthesia, the patient received the following regimen.
Antinociception:
- Remifentanil: 0.05 to 0.2 mcg/kg per minute
- Ketamine: 5 mcg/kg per minute
- Dexmedetomidine: 0.2 mcg/kg per hour
- Bilateral paravertebral block using 0.5% bupivacaine with epinephrine 1:400,000
Unconsciousness:
- Propofol: 30 to 100 mcg/kg per minute
Muscle Relaxation:
- Rocuronium: 10- to 30-mg bolus doses as needed
Hemodynamic Control:
- Phenylephrine: 10 mcg per minute after intubation
- Ephedrine: 5-mg bolus after intubation
We used the following management strategy during the case to titrate the anesthetics to a patient-specific level of antinociception and unconsciousness. The dexmedetomidine and ketamine infusions were used to create a background level of antinociception. For this reason, infusion rates of these anesthetics were kept essentially unchanged during surgery. The remifentanil infusion was used to maintain a background of antinociceptive control and additionally respond to acute changes in nociceptive stimulation during the surgery. If the patient’s level of unconsciousness was stable, that is, the EEG showed a pattern consistent with the patient being unconscious, then we decreased the propofol infusion rate by 10 to 20 mcg/kg per minute. If the decrease in the propofol infusion rate produced a concomitant decrease in the amplitude of the slow oscillations and/or a shift in the alpha power to the beta power range, then this suggested a decrease in the level of unconsciousness and the infusion rate was turned back up. This approach works to establish the lowest infusion rate of propofol that maintains a stable level of unconsciousness for the patient.
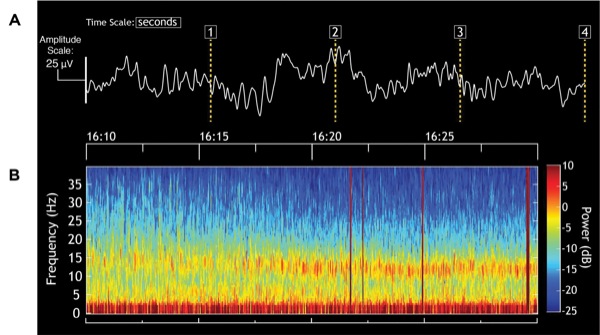
During this case, the level of unconsciousness remained stable. The EEG showed alpha (10-20 Hz) oscillations with slow-delta (0.1-4 Hz) oscillations in both the time-domain trace of the EEG (Figure 1A) and spectrogram (Figure 1B). From 16:10 to approximately 16:17, the power in the alpha band is at the higher frequencies of 15 to 20 Hz instead of the more common 8 to 12 Hz, as seen typically with propofol. This is due to the compromise frequency band created by the simultaneous infusion of propofol and ketamine. Propofol, in young adults, produces an alpha oscillation typically in the 8 to 12 Hz range.7,8 When administered alone, a low-dose infusion of ketamine produces a 25- to 30-Hz oscillation.7 When a propofol infusion (or an inhaled ether) and a low-dose ketamine infusion are administered together, they produce compromise frequencies between approximately 12 and 30 Hz, as seen here.9 The patient was hemodynamically stable throughout the procedure (Figure 2), suggesting that an adequate level of nociceptive control was maintained throughout the case.
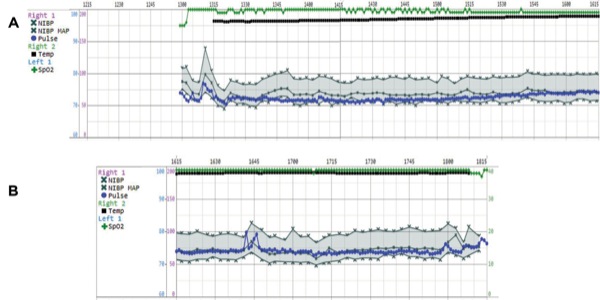
For emergence, the dexmedetomidine and ketamine infusions were discontinued approximately 20 and 30 minutes, respectively, prior to the end of the surgery. The propofol and remifentanil infusions were gradually decreased as the surgeons completed the last parts of the operation. At approximately two to three minutes after the infusions were stopped, the patient was following commands and was successfully extubated.
In the immediate postoperative period, the patient’s pain was managed with a single IV dose of hydromorphone. The patient received oral acetaminophen and oxycodone the morning following surgery.
Case 2
A woman who was at least 89 years old with a history of diabetes, hypertension and aortic insufficiency, and had a previous rodding of her left femur, underwent a laparoscopic right colectomy. Her weight was 57 kg and height was 153 cm. General anesthesia was induced with IV administration of propofol 100 mg, lidocaine 50 mg, fentanyl 100 mcg, and rocuronium 40 mg.
For maintenance of general anesthesia, the patient received the following regimen.
Antinociception:
- Dexmedetomidine: 0.2 to 0.5 mcg/kg per hour
- Remifentanil: 0.05 to 0.1 mcg/kg per minute
- Lidocaine: 2 mg/kg per hour
- Hydromorphone: 0.5 mg approximately one hour before the end of surgery
Unconsciousness:
Propofol: 30 to 100 mcg/kg per minute
Muscle Relaxation:
Rocuronium: 10-mg bolus doses as needed
Hemodynamic Management:
- Hydralazine: 10-mg bolus x 3 during induction
- Ephedrine: 5- to 10-mg boluses twice during maintenance
- Phenylephrine: 10 to 50 mcg per minute during maintenance
- Nitroglycerin: 50 to 100 mg per minute during emergence
The same strategy for maintenance of antinociception and unconsciousness used in Case 1 was also used for this case. In this particular case, IV lidocaine was used instead of ketamine as an antinociceptive adjunct, along with dexmedetomidine.
The EEG recordings (Figure 3A) and the EEG spectrogram (Figure 3B) suggest that an adequate level of unconsciousness was well maintained during the case. In particular, this patient shows EEG patterns that are common in elderly patients.10 The EEG is dominated by slow-delta and theta (4-8 Hz) oscillations. It is quite common to see either a decreased amplitude in alpha oscillations in elderly patients or, as shown here, a near absence of these oscillations when elderly patients receive a propofol infusion. Because the amplitude of the slow-delta oscillations can also be small, it is important for viewing these dynamics to be sure that the EEG traces are displayed on an appropriate scale. If the dynamic range of the scale on the EEG monitor is too wide, then the low-amplitude, slow-delta oscillations may appear as an awake pattern. Hence, the y-axis on the monitor scale should be decreased.
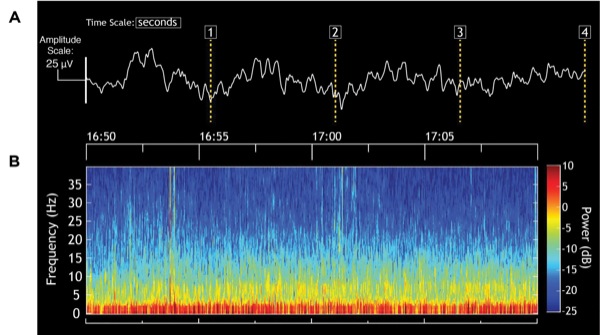
The patient required different cardiovascular medications for blood pressure control seemingly due to her poorly controlled hypertension (Figure 4). To reduce blood pressure, we used agents such as hydralazine during induction and a nitroglycerin infusion during emergence rather than propofol because the EEG showed a sufficiently adequate level of unconsciousness. During induction, the increase in blood pressure was treated with hydralazine and by starting the remifentanil infusion at 0.1 mcg/kg per minute and the dexmedetomidine infusion at 0.5 mcg/kg per hour, with the presumption that the increase was caused by not only the patient’s hypertensive tendency but also the nociceptive stimulation of intubation.

For emergence, the dexmedetomidine and lidocaine infusions were discontinued approximately 110 and 35 minutes, respectively, prior to the end of surgery. The propofol and remifentanil infusions were gradually decreased as the surgeons completed the last stages of the operation. The patient immediately gained consciousness and was extubated shortly after the infusions were stopped.
For postoperative pain management, the patient received acetaminophen the morning after surgery.
Case 3.
A 28-year-old woman with a ureteral stricture underwent a ureteral resection and reimplantation. Her weight was 95.3 kg and height was 162 cm. General anesthesia was induced with IV administration of propofol 200 mg, lidocaine 60 mg, and rocuronium 80 mg. A sufentanil infusion was started at 0.5 mcg/kg per hour at the beginning of induction.
For maintenance of general anesthesia, the patient received the following regimen:
Antinociception:
- Dexmedetomidine: 0.3 mcg/kg per hour
- Sufentanil: 0.1 to 0.5 mcg/kg per hour
- Lidocaine: 2 mg/kg per hour
Unconsciousness:
Propofol 30 to 100 mcg/kg per minute
Muscle Relaxation:
Rocuronium: 10- to 20-mg bolus doses, as needed
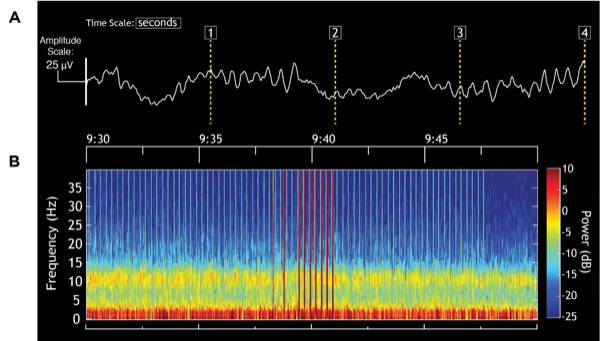
We used the same strategy for maintenance of antinociception and unconsciousness used in Case 1, except in this case, we used sufentanil instead of remifentanil to manage acute changes in the level of intraoperative nociception. Similarly, the pattern of alpha and slow-delta oscillations on the EEG showed that the level of unconsciousness was likewise well maintained (Figure 5A). The unprocessed EEG shows that the alpha oscillations were modulated by the slow-delta oscillation (Figure 5B). This peak-max pattern, meaning that the amplitude of the alpha oscillations is maximal at the peak of the slow-delta oscillations, is consistent with a state of profound unconsciousness.8 As the dose of propofol increases, this pattern devolves into burst suppression. The peak-max intervals become the bursts and the low-amplitude intervals become the suppression periods. The stable hemodynamics suggest that nociception was well controlled (Figure 6).

We discontinued the sufentanil, dexmedetomidine and lidocaine infusions approximately 30, 30 and 15 minutes, respectively, prior to the end of surgery. We discontinued the sufentanil infusion earlier than we would have stopped a remifentanil infusion because of the longer elimination time of the former drug. An added advantage is that unlike remifentanil, sufentanil contributes to the patient’s postoperative analgesia regimen. We gradually decreased the infusion rate of propofol as the surgeons completed the final parts of the operation. When the dressing was placed on the wound, the patient was awake and clearly following commands. Consequently, we were able to extubate her immediately.
The patient did not require pain medication until approximately five hours after the end of the case, when she received a dose of IV oxycodone.
Conclusion
Proper management of multimodal general anesthesia requires a shift in the conceptual framework used to infer the anesthetic states and make decisions about anesthetic drug dosing. The anesthesia care provider must have a strategy for monitoring level of unconsciousness and for monitoring level of antinociception. As described in the case studies, we use the real-time display of the unprocessed EEG recordings and its spectrogram to infer the patient’s level of unconsciousness. We have found this paradigm to be a more reliable way to track unconsciousness than use of EEG-derived indexes such as the bispectral index (BIS) or the Physiological State Index (PSI).11,12
Our use of the unprocessed EEG and spectrogram is predicated on the idea that maintenance of extra-cellular current oscillations is a primary mechanism through which anesthetics work.7,8,13-18These oscillations, which are readily visible on the EEG, impede communication among critical brain regions such as the brainstem, thalamus and cortex, leading to altered states of arousal such as unconsciousness.7,8,13-18 The oscillations change systematically with anesthetic dose, anesthetic drug class and patient age.7,10,19,20 Anesthesia providers using this paradigm take all of these factors into account when using the unprocessed EEG and its spectrogram to infer level of unconsciousness. This is in contrast to EEG-based indices such as the BIS and PSI, which oversimplify this information into a single number.
As mentioned above, we use changes in heart rate and blood pressure to infer level of antinociception. Use of heart rate and blood pressure in this manner is a long-standing, central feature of anesthesiology practice. It is justified by the nociceptive medullary autonomic (NMA) circuit, which is the most used pathway in anesthesiology practice (Figure 7). The NMA circuit governs how a noxious stimulus (e.g., a skin incision) in the periphery is transmitted via the dorsal root ganglia to projection neurons in the anterior lateral fasciculus of the spinal cord, which in turn synapse in the nucleus of the tractus solitarius (NTS) in the medulla.6 The NTS mediates efferent sympathetic responses through the ventral lateral medulla and the sympathetic ganglia in the spinal cord, which project to the heart and arterial blood vessels. At the same time, the NTS mediates the parasympathetic response through the nucleus ambiguous, which projects to the sino-atrial node of the heart.
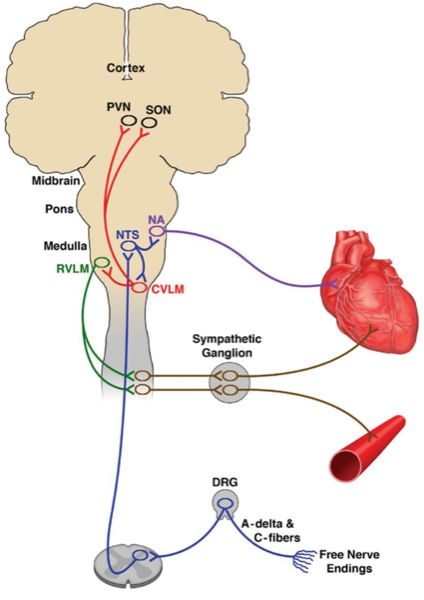
Use of the NMA pathway for inferring the nociceptive states requires taking account of the hemodynamic, respiratory and hematologic states of the patient, which clearly can alter heart rate and blood pressure for non-nociceptive reasons. Monitors designed to track heart rate dynamics and possibly electrodermal activity in real time to measure nociception are now available, and eventually may provide for measuring antinociception, what the EEG and its spectrogram provide for measuring level of unconsciousness.22,23 Muscle relaxation can be tracked by train-of-four monitoring along with the electromyogram displayed on some EEG monitors. The current strategies for monitoring antinociception, unconsciousness and immobility will no doubt undergo further refinements and improvements as multimodal practice becomes more widespread.
Because multimodal general anesthesia requires inferring in real time the states of antinociception, level of unconsciousness and muscle relaxation, this practice moves anesthesia caregivers away from one-dimensional concepts such as depth of anesthesia,23 minimal alveolar concentration (MAC)24 and target-controlled infusion (TCI).25 As pointed out several years ago, the depth of anesthesia is a misleading concept as it makes it appear that the ensemble of behavioral states required to produce general anesthesia can be compressed into a single dimension.26 Stated differently, use of multimodal general anesthesia makes explicit the behavioral states that the concept of depth of anesthesia oversimplifies. MAC and TCI provide strategies for anesthetic dosing in individual patients based on characterizations of population behaviors. Although the drug doses suggested by age-adjusted MAC and the infusion rates suggested by TCI offer plausible starting points to begin anesthetic administration, they can be rapidly adjusted in real time by tracking the patient’s levels of antinociception, unconsciousness and immobility.
In all of our examples, we used opioids (remifentanil and sufentanil) as part of the antinociception regimen during maintenance. In our experience, the use of one of these agents to respond to acute changes in nociceptive stimulation is very effective. Ketamine or fentanyl boluses also can be used to respond to acute nociceptive events. We use lidocaine and dexmedetomidine infusions primarily as adjuncts to create a background level of antinociception. The multimodal strategy obviates sole dependence on opioids.
We realize that some practitioners use all nonopioid agents to control nociception in their multimodal general anesthesia regimens to create a truly opioid-free management strategy.28 The antinociceptive strategy can also include regional techniques such as epidural infusions of local anesthetics, a transverse abdominis plane (TAP) block or paravertebral block. This was illustrated in Case 1, where a bilateral paravertebral block was administered as part of the antinociceptive regimen prior to induction. When regional blocks are used, we forgo lidocaine infusions to avoid any likelihood of local anesthetic toxicity. Our multimodal general anesthesia strategy provides a principled approach to reduce reliance on opioids by administering nonopioid agents following a neurophysiologically based strategy to effectively control nociception and pain.
While we exploit a priori the important secondary effects that the antinociceptive agents have on arousal, the anesthesia care provider must be cognizant of the other secondary effects of these agents when using a multimodal approach. For example, magnesium can promote hypotension, muscle relaxation and cardiac conduction abnormalities. Similarly, remifentanil and dexmedetomidine can promote hypotension and bradycardia.
The multimodal strategy for antinociception should be continued in the immediate postoperative period and following hospital discharge to manage pain. Use of multimodal general anesthesia will likely direct anesthesiology toward more personalized anesthesia care. This move will be guided by a conceptual framework that explicitly uses the definition of general anesthesia and places primary emphasis on nociceptive control to decide how anesthetics should be chosen and administered (Table 1).
References
- Brown EN, Lydic R, Schiff ND. General anesthesia, sleep, and coma. N Engl J Med. 2010;363(27):2638-2650.
- Brown EN, Pavone KJ, Naranjo M. Multimodal general anesthesia: theory and practice. Anesth Analg. 2018;127(5):1246-1258.
- Lundy JS. Balanced anesthesia. Minn Med. 1929:399-404.
- Hendrickx JFA, Eger EII, Sonner JM, et al. Is synergy the rule? A review of anesthetic interactions producing hypnosis and immobility. Anesth Analg. 2008;107(2):494-506.
- Tonner PH. Balanced anaesthesia today. Best Pract Res Clin Anaesthesiol. 2005;19(3):475-484.
- Reshef ER, Schiff ND, Brown EN. A neurologic examination for anesthesiologists: assessing arousal level during induction, maintenance, and emergence. Anesthesiology. 2019;130(3):462-471.
- Purdon PL, Sampson A, Pavone KJ, et al. Clinical electroencephalography for anesthesiologists: Part I: background and basic signatures. Anesthesiology. 2015;123(4):937-960.
- Purdon PL, Pierce ET, Mukamel EA, et al. Electroencephalogram signatures of loss and recovery of consciousness from propofol. Proc Natl Acad Sci U S A. 2013;110(12):E1142-E1151.
- Akeju O, Hamilos AE, Song AH, et al. GABAA circuit mechanisms are associated with ether anesthesia-induced unconsciousness. Clin Neurophysiol. 2016;127(6):2472-2481.
- Purdon PL, Pavone KJ, Akeju O, et al. The ageing brain: age-dependent changes in the electroencephalogram during propofol and sevoflurane general anaesthesia. Br J Anaesth. 2015;115(suppl 1):i46-i57.
- Kelley SD. Monitoring Consciousness: Using the Bispectral Index. 2nd ed. Boulder, CO: Covidien; 2010.
- Drover D, Ortega HR. Patient state index. Best Pract Res Clin Anaesthesiol. 2006;20(1):121-128.
- McCarthy MM, Brown EN, Kopell N. Potential network mechanisms mediating electroencephalographic beta rhythm changes during propofol-induced paradoxical excitation. J Neurosci. 2008;28(50):13488-13504.
- Ching S, Cimenser A, Purdon PL, et al. Thalamocortical model for a propofol-induced alpha-rhythm associated with loss of consciousness. Proc Natl Acad Sci U S A. 2010;107(52):22665-22670.
- Ching S, Purdon PL, Vijayan S, et al. A neurophysiological-metabolic model for burst suppression. Proc Natl Acad Sci U S A.2012;109(8):3095-3100.
- Flores FJ, Hartnack KE, Fath AB, et al. Thalamocortical synchronization during induction and emergence from propofol-induced unconsciousness. Proc Natl Acad Sci U S A. 2017;114(32):E6660-E6668.
- Soplata AE, McCarthy MM, Sherfey J, et al. Thalamocortical control of propofol phase-amplitude coupling. PLoS Comput Biol.2017;13(12):e1005879.
- Vijayan S, Ching S, Purdon PL, et al. Thalamocortical mechanisms for the anteriorization of alpha rhythms during propofol-induced unconsciousness. J Neurosci. 2013;33(27):11070-11075.
- Cornelissen L, Kim SE, Purdon PL, et al. Age-dependent electroencephalogram (EEG) patterns during sevoflurane general anesthesia in infants. eLife. 2015;4:e06513.
- Lee JM, Akeju O, Terzakis K, et al. A prospective study of age-dependent changes in propofol-induced electroencephalogram oscillations in children. Anesthesiology. 2017;127(2):293-306.
- Prys-Roberts C. Anaesthesia: a practical or impractical construct? Br J Anaesth. 1987;59(11):1341-1345.
- Dundar N, Kus A, Gurkan Y, et al. Analgesia nociception index (ani) monitoring in patients with thoracic paravertebral block: a randomized controlled study. J Clin Monit Comput. 2018;32(3):481-486.
- Ledowski T, Pascoe E, Ang B, et al. Monitoring of intra-operative nociception: skin conductance and surgical stress index versus stress hormone plasma levels. Anaesthesia. 2010;65(10):1001-1006.
- Palanca BJ, Mashour GA, Avidan MS. Processed electroencephalogram in depth of anesthesia monitoring. Curr Opin Anaesthesiol. 2009;22(5):553-559.
- Eger EI 2nd. Age, minimum alveolar anesthetic concentration, and minimum alveolar anesthetic concentration-awake. Anesth Analg. 2001;93(4):947-953.
- Struys MM, De Smet T, Glen JI, et al. The history of target-controlled infusion. Anesth Analg. 2016;122(1):56-69.
- Sleigh JW. Depth of anesthesia: perhaps the patient isn’t a submarine. Anesthesiology. 2011;115(6):1149-1150.
- Mulier J, Dekock M. Opioid free general anesthesia, a new paradigm? Best Pract Res Clin Anaesthesiol. 2017;31(4):441-443.
Leave a Reply
You must be logged in to post a comment.